Proteins are complex and fickle molecules. Experimental structure determination can teach us a lot about their function, but this is not the easiest thing to do. It’s not as simple as looking through a microscope, focussing, and taking a picture of the protein. It’s more like when you have a broken arm and the doctor uses X-rays to see what is inside, with the minor caveat that you first need to remove your arm from the rest of your limbs, crystallise your arm with thousands of other identical copies, and THEN shoot the high energy X-rays at the crystal. After successfully navigating a whole lot of maths, and you can finally get a protein structure.
Different Methods
Experimentally determined corona virus protein structures can come from three sources: X-ray crystallography, electron cryo microscopy (cryo-EM) and solution nuclear magnetic resonance (NMR). Each experimental technique has its own advantages and disadvantages. These structural techniques can also be complemented with a number of other techniques such as mass spectrometry, chemical cross-linking, fluorescence resonance energy transfer, and genetics, to fill in the finer details around structure and function.
Cryo-EM
If you are looking to find the structure of a “big” molecule (although it's still quite small, really) electron microscopy can help you. Unlike in other techniques, the biomolecule of interest is imaged directly using a beam of electrons and a system of lenses. The complicated bit is turning these 2D pictures into 3D objects. This can be achieved by imaging thousands of the same kind of biomolecules in different orientations, so that we can reconstruct it in 3D. Although Cryo-EM is historically considered a lower resolution technique, recent technological advances have brought about a resolution revolution, and some structures are almost as detailed as those from X-ray crystallography. As a result, Cryo-EM can now show us amino acid sidechains, surface water molecules, and non-covalently bound ligands, which were previously the purview of X-ray Crystallography alone.
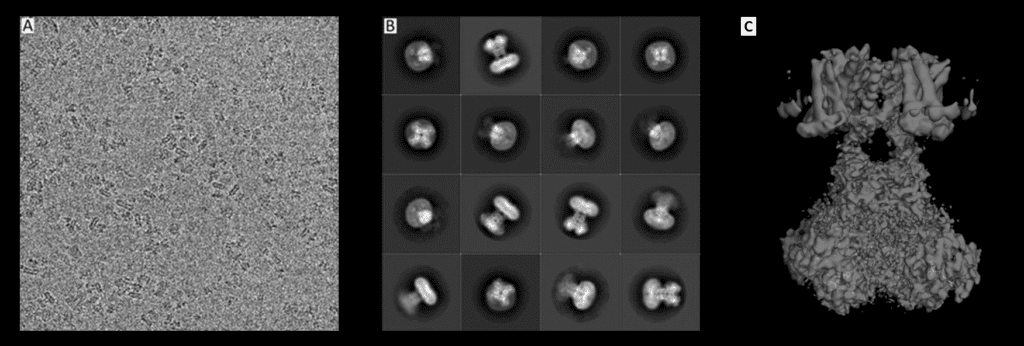
Original pictures from: Matthies, D., Bae, C., Toombes, G.E., Fox, T., Bartesaghi, A., Subramaniam, S., Swartz, K.J. (2018) Life 2018;7:e37558, edited by Ferdinand Kirsten, License: CC BY-ND 2.0
NMR-spectroscopy
An important step in NMR-spectroscopy is the so-called isotope enrichment. While the typical MRI at your doctors just measures the basic whereabouts of the atom nuclei in a certain tissue, this method can help identify the distribution of carbon atoms in a structure. However, this requires that some carbons must differ from others. Different isotopes of carbon, with different numbers of neutrons in their nuclei, are incorporated into the protein in the purification process. After this purification, the protein is suspended in a strong magnetic field and is probed with radio waves. The distinctive resonance of each isotope is then analysed, yielding information about the whereabouts of the different carbon nuclei and revealing the distances and possible connections between them. Using the knowledge about these distances, scientists can solve the Sudoku-like puzzle to generate an atomic model of the protein. This method only works for small to medium sized proteins, as larger structures cause problems with overlapping peaks in the resonance spectra. On the other hand, NMR-spectroscopy has a major advantage in its ability to measure flexible proteins in solution instead of solid states, which may hinder molecular movement.
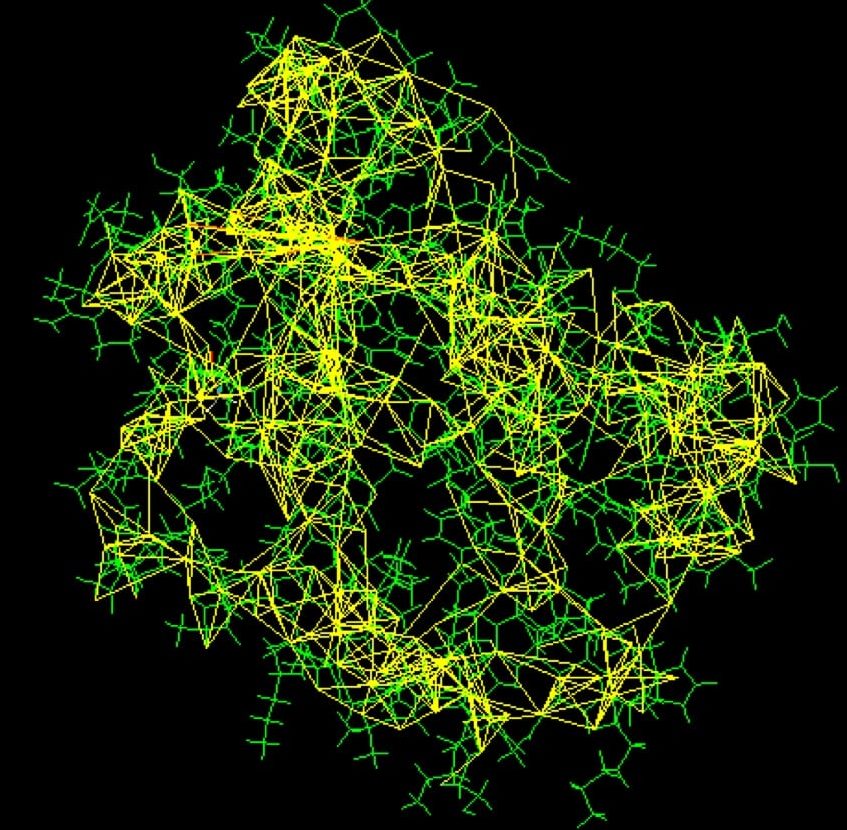
Picture courtesy of PDB101.rcsb.org
X-ray crystallopraphy
Of the methods discussed here, X-ray crystallography has produced the most structures to date, totalling 145 252 in the PDB compared to 12 965 from NMR and only 4 926 from Cryo-EM. However, X-ray crystallography has a major drawback: the need for a protein crystal. While this common method can provide very detailed atomic information, showing every atom of each amino acid and even of ligands, inhibitors, ions and other molecules included in the structure, the process of crystallization is difficult and might limit which type of protein that is studied. Purifying a protein for crystallisation has become much more straightforward in recent years but is still a non-trivial task. After purification, the production of a protein crystal from can take months to years before structure-worthy data can be measured from it. In particular flexible proteins are much harder to crystallise. As enzymes or receptors, a lot of proteins rely on movable parts and different conformations to fully operate, so unfortunately, interesting proteins are often flexible! Once produced, the crystal is cooled in liquid nitrogen and subjected to an intense X-ray beam. You can compare this to a cystal that you hold to the light and observe its reflections on a wall. In this case, the X-rays hit the protein and get diffracted in a specific pattern, but the diffracted rays cannot give a picture of the crystal, but need to be interpreted with a structural model. The distribution of electrons can be calculated from this pattern, resulting in an electron density map with the estimated location of each atom.
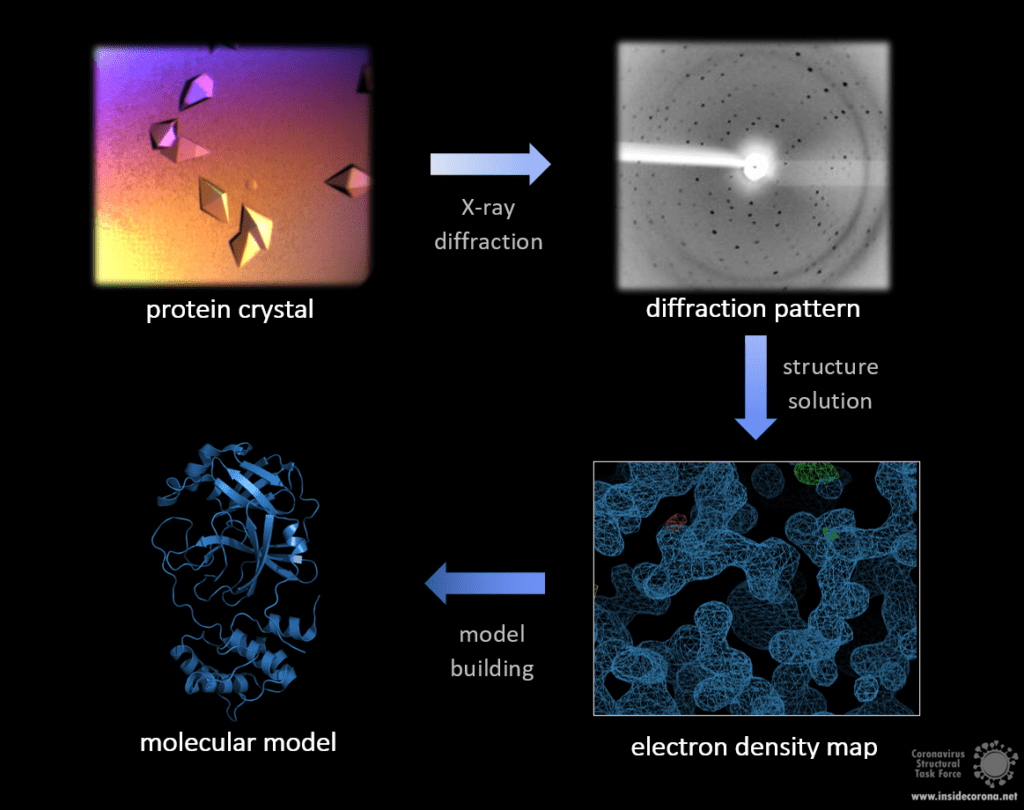
Crystal by Andrea Thorn, Diffraction pattern by Sabrina Stäb, image by Ferdinand Kirsten.
Prospect
The molecular models obtained by these methods open up numerous possibilities: structure-based drug design, computational dynamics simulations and answers to biological questions. But how can we interpret and refine those models to extract every last biological detail? This will be discussed in the next blog entry.
Interested? Learn more about it:
- https://pdb101.rcsb.org/learn/guide-to-understanding-pdb-data/methods-for-determining-structure
- https://proteopedia.org/wiki/index.php/Resolution
- https://www.ncbi.nlm.nih.gov/pmc/articles/PMC4465431/
- https://pdb101.rcsb.org/learn/guide-to-understanding-pdb-data/r-value-and-r-free
- https://pdb101.rcsb.org/learn/guide-to-understanding-pdb-data/biological-assemblies
- https://www.rcsb.org/stats/summary